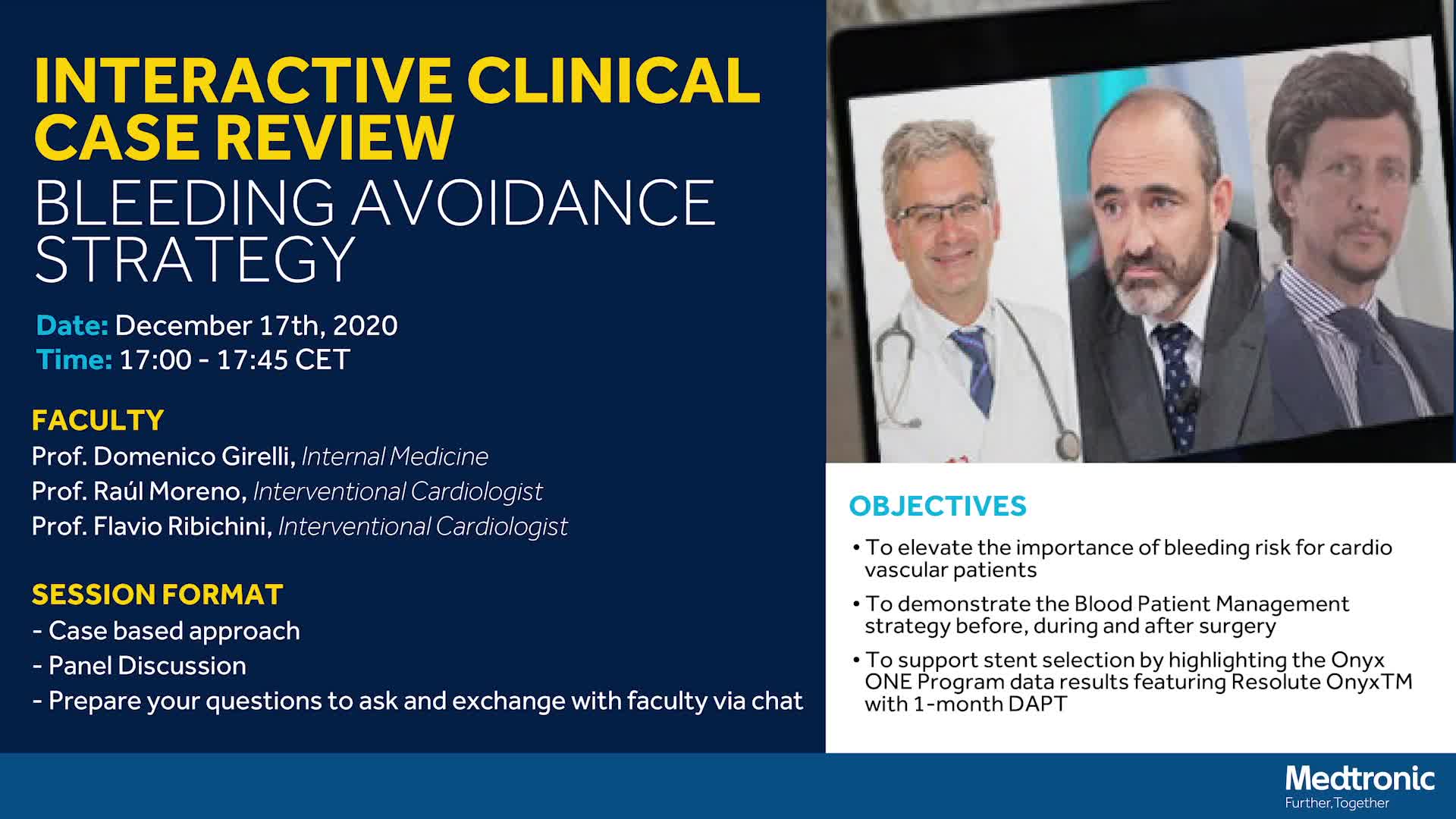
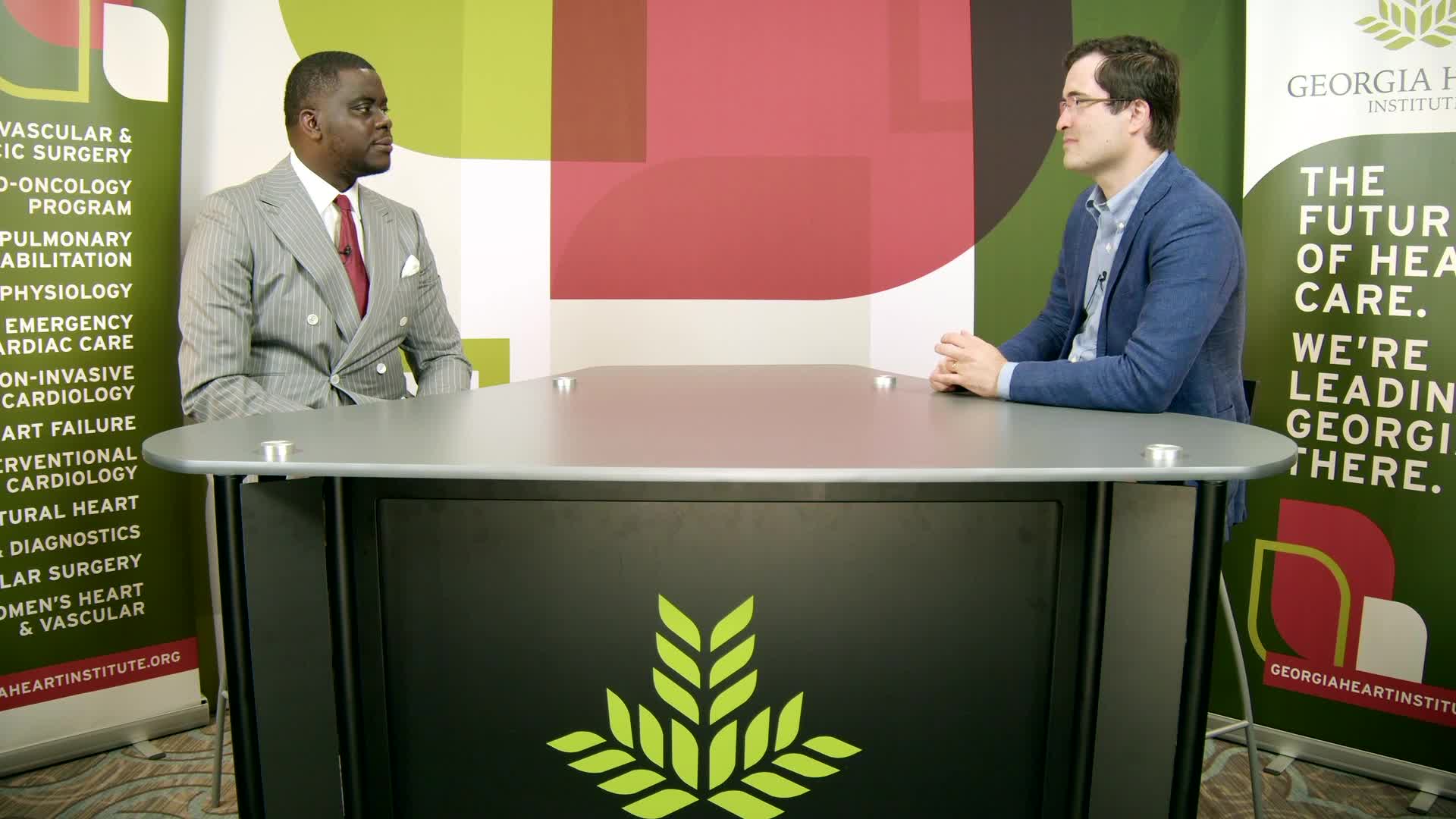
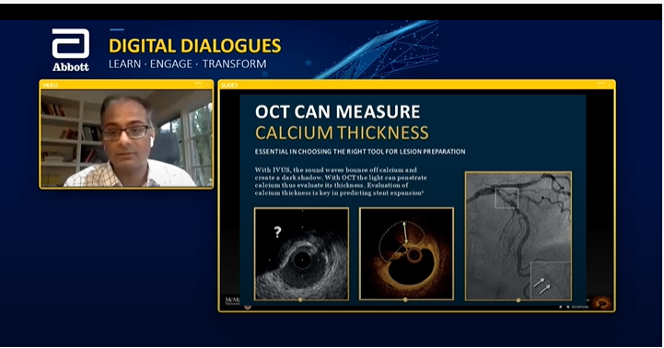
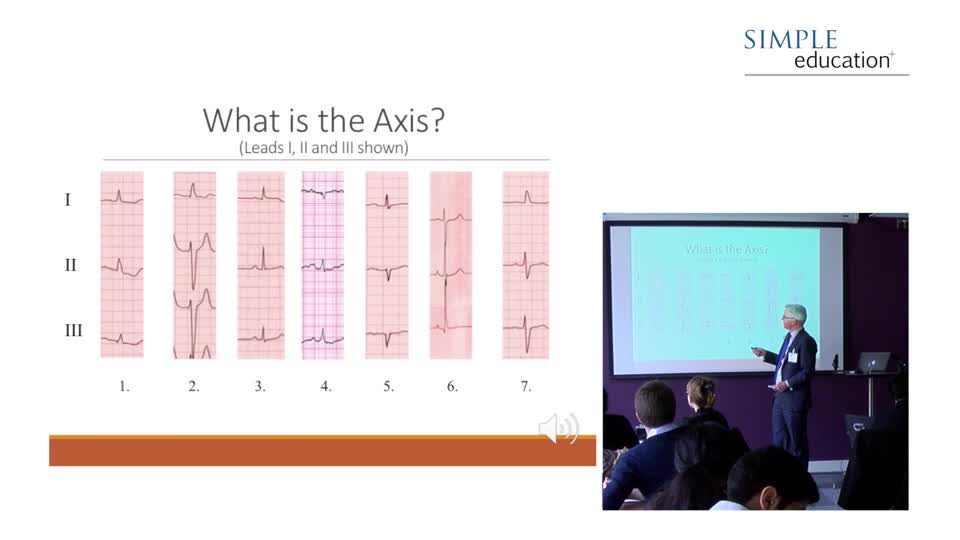
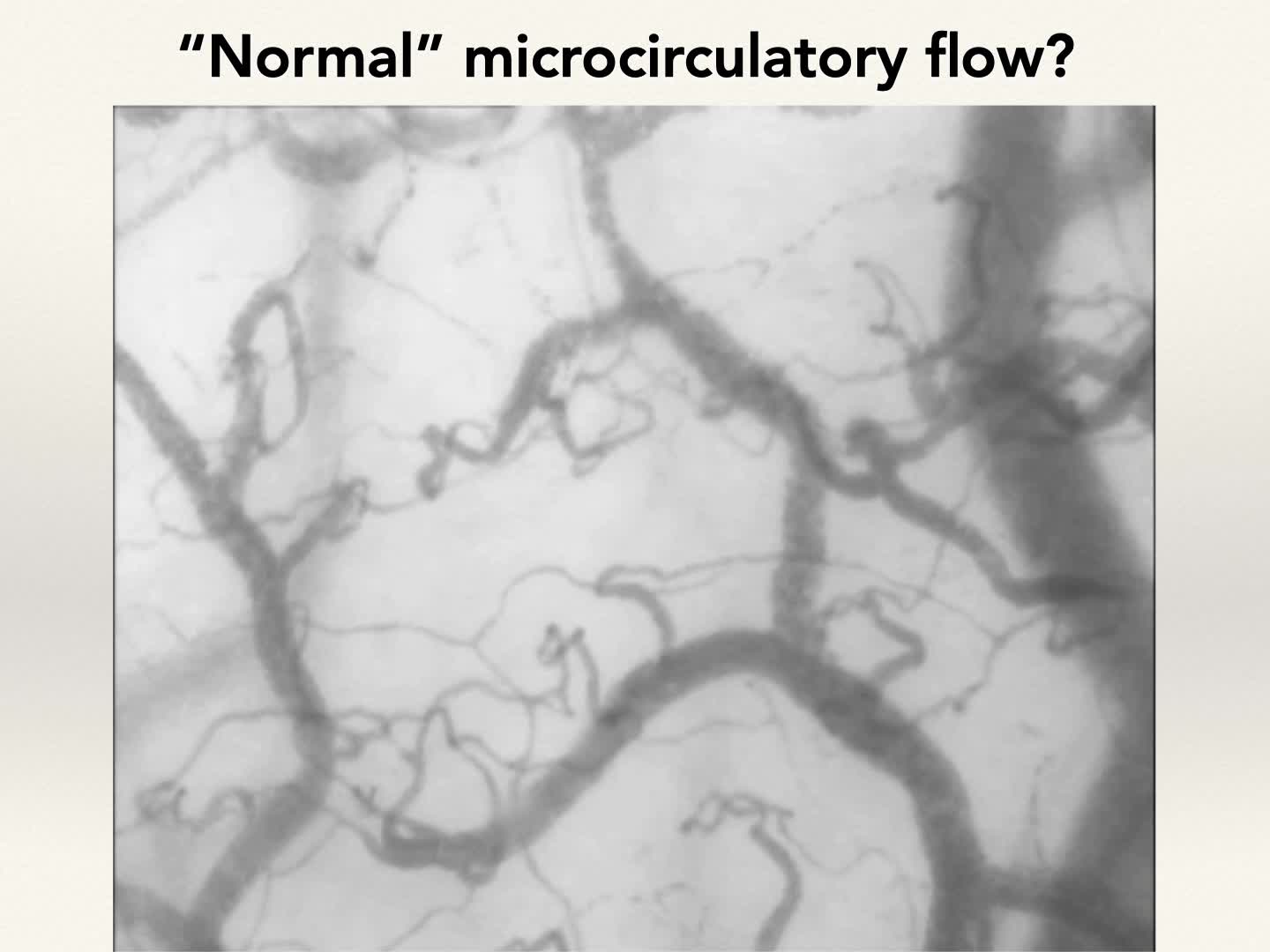
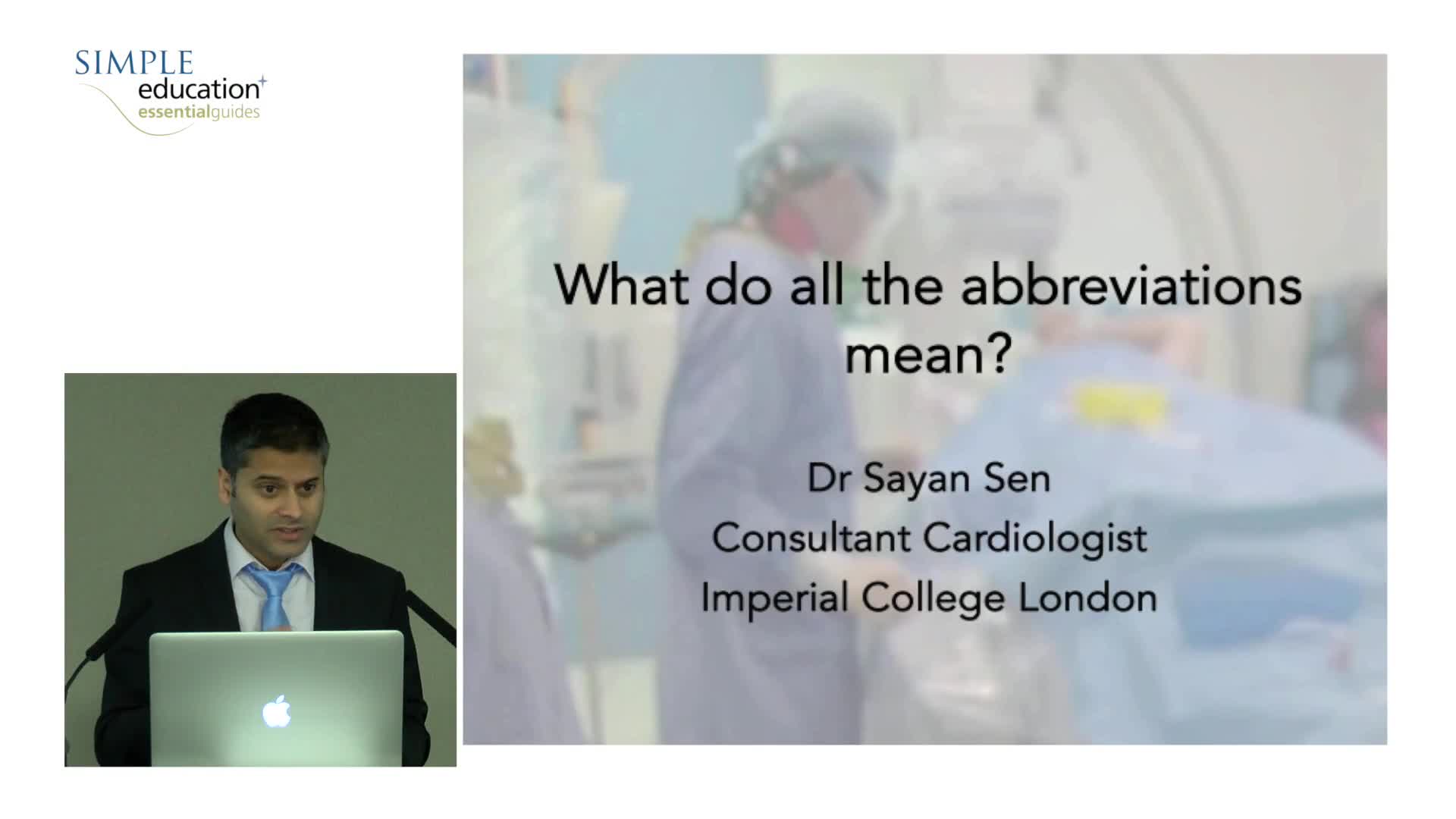
Managing Acute Respiratory Distress Syndrome (ARDS) in COVID-19.
So, we're now going to carry on with our talk on Acute Respiratory Distress Syndrome (ARDS). And we're going to look at the long-term outcomes of those patients that survive.
So, here is a table of...
So, we're now going to carry on with our talk on Acute Respiratory Distress Syndrome (ARDS). And we're going to look at the long-term outcomes of those patients that survive.
So, here is a table of the pulmonary function tests of those survivors 3, 6, and 12 months after discharge. And you can see they're not quite normal. But the one I'd like you to focus on is the DLCO. That's the diffusing capacity for carbon monoxide, which best correlates with your lungs' ability to transfer oxygen. And you could see, at 12 months, it's still far from normal in fact. But, most importantly, is what can you actually do? And if you see the test that we normally use, it' is called a six minute walk test, and that tells you really how much functional recovery you've made. And at 12 months you can see you're still quite a ways from normal, about 60% of normal.
The surprising aspect that's been talked about and it's been investigated quite a bit recently is cognitive impairment. And what's interesting is that recent neuroimaging has demonstrated that the functional impairment is married to the anatomical damage. And you can see this with MRIs. And these abnormalities can persist for months to years after discharge ITU. And it's seen with global and focal atrophy. And, in fact, up to 50 or more percent of survivors had cognitive impairment, well, up to a year and after, in fact. And this impairment was comparable to a moderate traumatic brain injury, or mild Alzheimer's disease. And that's quite, quite surprising.
This is an MRI of somebody who is a normal brain, and you can see the lateral ventricles are normal size. And about a year or two later, you can see that they have expanded signifying cerebral atrophy.
Now, a woman named Margaret Herridge, a Canadian doctor has been, probably the world's leading authority on outcomes after ARDS. And in 2003, she published this study of survivors of ARDS. And you can see that they were looking at the clinical signs 3, 6, 12 months after discharge. And they looked at symptoms, pulmonary function tests, quality of life, and return to work or not.
And this is where the outcomes looked like. And by the way, there was a recent 2020 article in CHEST that confirmed everything that was seen in 2003. So, what did they find? Fatigue. Almost two thirds of patients who survived were fatigued at one year, serious and handicapping fatigue. Weakness that was not respiratory was seen. Quality of life scores were poor. And only about 50% of those that were working beforehand actually returned to work.
Now, what's the cause of this? Well, could be steroid myopathy because we do give steroids to these patients quite often. Neuromuscular blockers, now that's a controversial statement because quite frequently we need neuromuscular blockers to allow patients to be ventilated because, if they have a very strong respiratory drive they will not accept ventilation from our ventilators. And so, we have to avoid dyssynchrony, and we have to use heavy dose of sedatives. And if they don't work, we use neuromuscular blockers. This is a little bit controversial, not everybody has found this.
Critical illness neuromyopathy. That's a common problem in those that have been in intensive care for any length of time. Disuse myopathy, and weight loss. Basically, the catabolic processes that break down proteins in your muscle will leave you feeling quite weak.
So, let's now talk about our goals. One thing we must not do is confuse our goals. Now, we talked about the P/F ratio as being a marker of the efficiency of a lung's ability to transfer oxygen from the gas we give it into the arterial blood. And it's a marker of the severity of ARDS. And we can use that to trend and see the efficiency over time of your lung injury.
So, now, let's look at how this P/F ratio works. So, here is the oxygen dissociation curve. And on the X axis, you have the PaO2 in kilopascals and the P/F ratio. On the Y axis, you have oxygen saturation. Now, someone who has perfectly normal lungs, who's receiving 100% oxygen, so that's an FiO2 of 1.0, may have a PaO2 of, say, 88. And 88 divided by 1, because it's a 100% oxygen they're receiving, means they have a P/F ratio of 88. And, of course, they'll have a saturation of 100%.
Now, you may have someone with very sick lungs, give them a 100% oxygen. And they may have a PaO2 of 27, which because of the 100% of oxygen they're receiving means they have a P/F ratio of 27, 27 divided by 1. And they too will have a saturation of 100%. But the big difference is that the ARDS patient, if they were to reduce, or their FiO2 is reduced, they would immediately slide down the saturation curve. Unlike the normal patient who has a large reserve. So, the P/F ratio really gives you an idea of how healthy or not your lungs are. And that's what we use to trend and follow patients. And you can see there's a massive difference in reserve.
Now, this may seem a bit surprising to some. Now, let's say you take a liter of arterialized blood. And you put it on the table, you take cardiac output out of the equation. So, we're talking about the oxygen content of this blood. And you look at someone who has a normal hemoglobin of, say, 15 and they have a normal saturation, and you'll see that they will have an oxygen content of 200 milliliters of oxygen per liter of blood. Now, let's say you take that same liter of blood and instead of having a hemoglobin of 15, you have a hemoglobin of 7.5, and your saturations are normal. You'll see that, mathematically, you have half the oxygen content. So, that's 100, excuse me. Now, let's say you have the same liter of blood, this time with a normal hemoglobin, and you have a saturation of 80%. Well, 80% of 200 is 160. So you can see that, in fact, the person who has the anemia, but with a normal saturation has quite a bit less oxygen content than the patient who has a normal hemoglobin, but an 80% saturation.
Now, ironically, in the wards, we see plenty of patients who have hemoglobins of 7 or so. And if they look okay, we leave them. On the other hand, if somebody had a saturation of 80%, I can guarantee you, no nurse would leave you in peace. Now, what worries me is not the 80% per se. Although, I'm not saying it's okay to leave someone with a saturation of 80%. It's just that I'd like to know why it's 80%. Now, this is just basically the physiology. And you have to bear in mind that not everything we do is always rational. We accept one, but not the other.
Now, this is the true story of a woman who was 21 years old. She was admitted to our unit with acute respiratory distress, following flu like symptoms from H1N1. She required intubation. Her oxygen saturations were low at 87, and they dropped at 78 quite rapidly, despite 100% oxygen. Now, her central venous saturation was 72%, which is normal. Now, we tried to improve her oxygen saturation with much higher PEEPs of up to 25 centimeters of water. The oxygen saturations didn't really improve much, but her central venous saturation, now, was 52% and that's very low.
So, why did this happen? What does this signify? And what are we going to do about this? Now, the reason I bring this up is because you've got to be very careful what our goals are. And our goals are not oxygenation, it's oxygen delivery. So, let's say, you have somebody who has a low P/F ratio, as you can see here, and you want to improve that. So, you increase your PEEP and as you increase your PEEP, you see that for the same FiO2, your P/F ratio is going to increase. And so, your oxygen saturation on the monitor is going to go up. And you're going, "Wow, fantastic. We've won here." What you may not notice is the cardiac index may, not always but may, drop. And that means, overall, your oxygen delivery may decrease. And, at the end of the day, what we care about is the oxygen delivery.
Now, referring back to that previous case, what we had probably done was by increasing the PEEP to try and improve the saturation, we took a central venous saturation that was relatively normal, and it now started dropping, which means we probably were reducing the cardiac output, and therefore reducing the oxygen delivery. And so, we were causing quite a bit of harm potentially.
Now, what do we mean by oxygen extraction? So, here's a capillary and there's a cell. We have arterial inflow and we have hemoglobin that's fully saturated, so all four sites are carrying oxygen, and therefore it's 100% saturated. Now, one of those oxygen molecules will be extracted, and will go to the cell, and it will be used to produce 36 ATPs. That's normal. And then, when it goes past the cell into the venous outflow, it will have three out of the four sites occupied with oxygen. And, hence, you'll have an oxygen saturation of 75%. Now, most tissues have slightly different metabolic rates. And so, when you take a sample from a central venous catheter in the superior vena cava, for example, it may be 70, 72. It's not always 75.
Now, what happens is if you don't have enough oxygen delivery to your cells, your cells have the capacity to temporarily extract a second molecule of oxygen. And so, now, the hemoglobin flowing back to your right heart will not be 70 or 75% saturated. It'll be lower, so maybe 60 or so. Now, what that signifies is that your cells are using their last mechanism to keep themselves functioning. And so, that's a temporizing maneuver, and it's not something you want to just sit back and watch. You want to make sure you have to do something about that. That's sort of your last gasp, if you like, and it's a temporizing measure.
So, to recap, mortality is decreasing with ARDS, but it's still deadly, about 30 to 40% of patients will die with it. We cannot make you better, we can't cure you, but we can definitely make you worse. Now, how would we do that? Well, if we overstretch your lungs. And overstretching means that we put a tidal volume that is too large for the size of your lung. And so, we've made a first important step in realizing that the tidal volumes we used to use were much too large for the normal lung capacity.
Today, we now recognize that the sick lungs are even smaller than your normal lung, and that's what they call baby lung. And so, we're trying to find ways of minimizing the excessive tidal volume we placed in those smaller lungs. That's for another lecture. But today, at least we don't want to use large tidal volumes. And so, we try not to overstretch the lungs.
Secondly, you don't want to blow up the balloon and then let it collapse down again. That's what we call recruitment and de-recruitment. that damages your alveoli. So, what do we do? We use "low," which are really normal tidal volumes. And to guess, or to estimate, or guesstimate what your normal tidal volume ought to be we have to calculate your ideal body weight. And it has nothing to do with your weight it's to do with your height and your sex. And we follow the efficiency of gas exchange using the P/F ratio. And that also allows us to stratify the severity of ARDS and therefore, marry the optimal treatment to the severity. So, let's not forget we are not in the oxygenation business, we're in the oxygen delivery business. And that means we have to keep an eye on cardiac output, hemoglobin, and oxygen saturation.
Now, given the recent COVID pandemic, we've seen some very interesting phenotypes, or expressions of this ARDS illness. And, in fact, what we see doesn't correspond to what you've been describing in this lecture. This is not your typical ARDS, at least to begin with. And so, Professor Gattinoni, who's probably the world's leading, or one of the world's leading authorities on ARDS has described two types of injury. A type 1 or a H for a high compliance. And what he finds here, and you see the CT, is relatively spared in terms of the aerated lung. So, the black bits are actually bits that are taking part in gas exchange. So, you can see the lung weights are just over 1,000 grams. The non-air aerated tissue is only 8.4%. So, most of the tissue is black hence, it is taking part in gas exchange.
The P/F ratio is very, very poor. The venous admixture is 56%. And, most importantly, the compliance is quite high. So, everything we talked about in terms of treatment, and PEEP, and recruitment was to try and improve a stiff noncompliant lung. And, in this case, it doesn't make sense to try and expand a lung that's already expanded.
The second type is your classic ARDS, your L or low compliance ARDS. And you can see that the weights are a bit higher but, most importantly, the non-aerated tissue is 39%. So, you have a lot less of the lung that's open for aeration and oxygenation. And the compliance here is 43, so it's a lot worse. It's considerably worse.
So, what does Gattinoni present as a treatment option? He says in the type 1 patient, in other words, high compliance, you want to keep the PEEP levels much lower. There's no point in trying to recruit a lung that's already open. You'd just cause more damage. You can probably get away with somewhat higher tidal volumes because the lung is somewhat supple, and that would help you with CO2 removal. So, he says going up to about 8 or so, mils per kilo, ideal body weight. You don't want to increase your respiratory rate too high because the repeated cycling of tidal volumes actually causes damage in itself. So, staying to around 20 breaths a minute is a pretty reasonable number. So, you want to basically keep things quiet. Don't overdo things, and be patient things will get better. Don't try and chase after some magic number.
The type 2 or L patient has a typical ARDS, the ones that would require the type of treatments we've talked about, whether that's lower tidal volumes, proning definitely, and higher PEEPs and recruitment maneuvers.
Now, I want to talk about one aspect of this that's been very bizarre. We have all seen patients who arrive, who are sitting, talking on their telephone. They don't look distressed, and yet they are very blue. They're very hypoxic. Now, why would that be? Well, you have to understand a few concepts first to understand what's happening. Normally, you marry your alvelolar unit, and your pulmonary capillary unit, so that you have adequate ventilation and adequate profusion, and that's called a normal VQ.
So, this is what a normal VQ looks like. Here's your alveolus getting good ventilation and it's got the normal oxygen and CO2. And there's your blood flow going past it to pick up oxygen and dump CO2. And it returns into the pulmonary vein with a normal oxygen saturation of 98%. That's how things ought to work.
Now, before I go on, I just want to say that normally your pulmonary artery can generate a systolic pressure, so a pulmonary systolic pressure of about 30. Compare that to the systemic pressure, which is about 120. And so, you're much lower pressures are going to be influenced by gravity. So, the apical regions, or the non-dependent regions of your lungs are going to be less well perfused compared to the dependent, or lower regions of your lungs. More like the basal regions of your lungs. And so, when you have a sick lung, you have some units that have got, what we call, high VQ.
What does that mean? That means you have a lot of ventilation and relatively poor perfusion. So, the marriage between the two is not perfect. And because the small quantity of blood traversing the alveolar unit is traversing a very well oxygenated unit it's fully saturated. And that's fine. But you'll have a large area of low VQ. What that means is you have poor ventilation, but you have a lot of perfusion. And that's probably much more common because of the gravitational aspect. So, you're having a lot of perfusion in areas that are relatively poorly ventilated when you have sick lungs. So, that's flow VQ. And because they're so poorly ventilated, you're going to come away with a saturation in your pulmonary vein, which is much lower. So, you'll have 99% saturated mixing with 80% saturated.
Now, you might think, "Okay so, the mean between the two would be around 90%." But, in fact, because there's so much more blood from the low VQ area, and you can see that by the thickness of those arrows, in fact it's not the arithmetic mean. And, in fact, it's going to be closer to the 80%. So, it may be closer to, say, 85% saturated. So, it's not going to be the arithmetic mean between the higher and lower saturations. It's going to be much more towards the low VQ, which drags it closer to its value.
Now, one thing that is interesting with COVID is normally what happens if you have a hypoxic alveolar pulmonary unit, you have something called hypoxic pulmonary vasoconstriction, or HPV. And what that does, it's like a faucet, you turn off the tap and so the blood will not be going to those poorly ventilated units. And the blood will be sort of forced into the higher ventilated units. So, that's exactly what you'd imagine would happen. And that's ideal. So, you're going to get better oxygenation where it's taking place. For some reason, with COVID, we seem to have lost that hypoxic pulmonary vasoconstriction.
Now, the reason is because their lungs are not stiff. And it's the stiffness that makes that extra work of breathing. And so, they don't look like they're breathing hard. They're not working hard, but they're talking on their telephones and they're blue, and that's why we call them happy hypoxics or silent hypoxics.
Now, the last thing is there is something called true shunt. And what that means is the blood that's perfusing doesn't come into contact whatsoever with oxygen. So, that's blood that's just passing through, and there is absolutely no contact with oxygen. So, how do you distinguish true shunt from a low VQ? Well, it's very easy, you give 100% oxygen. And with a low VQ unit, if that's the majority of those units, then you'll see a rise in your PaO2 because even though there's not a lot of ventilation going in and out that which has taken place has got a lot of oxygen in it. And so, that will raise your PO2. If you have a pure shunt, it doesn't matter how high the oxygen and your alveolus is none of it's going into contact with the blood that's being shunted.
And, finally, you may ask why a low cardiac output may cause or exacerbate hypoxemia? And this is often an aspect of care that we neglect. So, a low cardiac output can make your hypoxemia worse. And we've often seen patrons where you improve the cardiac output. And by probably improving the mix of venous or central venous saturation, you're actually reducing the hypoxemia.
Now, how would that work? Well, the oxygen in arterial blood represents the sum of the oxygen in mixed venous blood, pulmonary artery blood, and the oxygen added from the alveolar gas. When gas exchange is normal the PaO2 in our alveolar gas is the major determinant of the arterial PO2. However, when gas exchange is impaired, the contribution of the alveolar PO2 declines and the contribution of the mixed venous PO2 rises. The greater the impairment of the gas exchange, the greater the contribution of the mixed venous PO2 to the arterial PO2.
And, finally, this recent article in The Lancet Infectious Diseases that just came out, looks at dual-energy CT. And you can see on the CT, there are areas of abnormal lung with the green arrows, and this is the blood flow demonstrating a loss of hypoxic pulmonary vasoconstriction, and hence a worsening of the VQ abnormalities. And that could explain the clinical hypoxia in what are relatively compliant lungs.
So, how can we tie all this together? A biologically plausible explanation is that initially the COVID lung is not a small baby lung, but an adult lung with near normal size and compliance, but with a major vascular problem. That is to say, loss of hypoxic pulmonary vasoconstriction leading to VQ abnormalities as previously described. Thus COVID, at least initially, is an atypical ARDS. This leads to your happy hypoxics that we've seen on the ward, who do not appear distressed, but in response to the hypoxia have a high respiratory drive that's being generated, and therefore have very large tidal volumes.
It must be understood that the lung takes a similar hammering, whether large tidal volumes are delivered from a ventilator, or by the patients themselves. This is a state called P-SILI, patient self-inflicted lung injury. So, over time if this continues, then the atypical ARDS becomes the more classic baby lung style ARDS described in the beginning of this talk, what some are calling a VILI vortex, hence the need for a very personalized approach which seems, to me, essential.
So, if you have any desire to see any of the references, you can go to my website and you have the username and password at the bottom. Thank you.